Thermoelectric Energy Generation Takes Flight for Aircraft and Spacecraft Monitoring
投稿人:DigiKey 欧洲编辑
2014-04-23
Military and aerospace, where rugged operation and reliable performance in a confined, hostile environment are paramount, have long been the most dominant markets for thermoelectric energy harvesting. Typically, thermoelectric devices exploit heat from engines and motors and use it to power sensors and wireless sensor networks for condition monitoring applications. Recent innovations are generating growth in this sector, as well as in allied sectors.
This article will review some of the major avionics and aerospace applications that use thermoelectric devices. For example, commercial and military aircraft incorporate sensors and sensor networks powered by thermoelectric generators to monitor the aircraft skin for damage that can cause stresses and structural weakness. In the aerospace sector, the Mars Rover, Curiosity, Galileo satellites, New Horizons space probes, and Cassini spacecraft are all TEG users.
Typical devices that will be considered include the CP range of TEGs from CUI Devices, and the eTEC modules from Laird Technologies. Further consideration will be given to the management of energy generated by TEGs, with reference to the LTC3108 DC/DC converter from Linear Technology.
Heat differential
The principle phenomenon that underpins thermoelectric generators (TEGs) is the generation of electrical energy by exploiting the temperature difference as heat flows between two materials. This is known as the Seebeck effect. The larger the differential, the more energy can be generated.
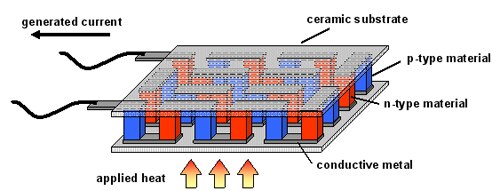
Figure 1: Structural diagram of thermoelectric module using the Peltier effect to generate energy from heat flow.
Using the reverse phenomenon, called the Peltier effect, thermoelectric coolers (TECs) produce a temperature differential by applying a voltage (see Figure 1). The polarity of the output voltage is dependent on the polarity of the temperature differential across the TEC. Reverse the hot and cold sides of the TEG and the output voltage changes polarity. A useful Application Note from Linear Technology explains these principles clearly.¹
In fact, TEGs or TECs can be used for heating or cooling applications, as well as for energy generation. There is no functional difference between modules used for either application, but rather their mode of usage differs, and sometimes their construction with respect to their electrical circuits.
Increasing market interest
Despite the recognition of the huge potential of thermoelectrics to reuse waste heat, harvesting energy using TECs and/or TEGs has seen limited application to date. Yet, the technique has been the object of extensive research, and it is only recently that developments have stimulated an increased interest.
The stimulus for research into TEGs can at least partly be attributed to the pervasiveness of wireless sensor networks and the need for autonomous operation. This is especially true in applications such as condition monitoring in industrial environments, smart metering, environmental monitoring in remote locations, structural monitoring, and in the transport sector in vehicles, aircraft, and spacecraft.
A US research team, comprising chemists, material scientists, and mechanical engineers from Northwestern University and Michigan State University, has developed a new, more efficient thermoelectric material based on the common semiconductor telluride.² Being environmentally stable, it is expected to convert from 15 to 20 percent of waste heat to electricity. The researchers believe that with a figure of merit, ZT, of 2.2 (compared to a more typical figure of 1), the new material is efficient enough to be used in practical military and industrial applications, prompting widespread use.
TEGs can be used autonomously to power wireless sensor networks, and are particularly in demand where there is no constant power source, where access for maintenance or changing a battery is impractical, and where other sources of ambient energy are not consistent. Indeed, TEGs are perfect for use in hostile environments such as in space. They use no liquids, thrive in high temperatures, and are not reliant on sunlight (like solar) or movement (piezoelectric).
TEGs can, of course, also be used in conjunction with an energy storage device, such as a supercapacitor or rechargeable battery to provide a more conditioned power source. The advent of extremely-low-power electronics, as used in wireless sensor networks, has given rise to a growing number of applications that operate with small temperature differentials, as low as just a few degrees.
According to market research company IDTechEx, the market for thermoelectric energy harvesters will reach $875 million by 2023³ representing significant growth over the next decade. Thermoelectric is now ahead of piezoelectric in terms of investment and commercialization, the market researchers maintain. Despite the growing interest from consumer and industrial sectors, customized military and aerospace applications dominate the market.
Space heater
TEGs have been used by US, and other, space programs for many years. Indeed, they have proved to be a safe, efficient and long-lasting power source for Apollo missions to the moon, the Viking missions to Mars, and the Pioneer, Voyager, Ulysses, Galileo and Cassini missions to the outer solar system. More recently, they have been used to provide energy for the Mars Rover, Curiosity.
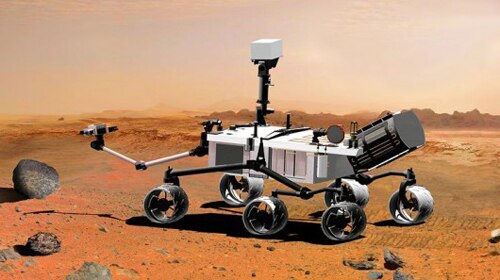
Figure 2: NASA’s Mars Rover, Curiosity, is powered by a TEG.
NASA chose to use a TEG power source because solar power alternatives did not meet the full range of the mission’s requirements. The controllers required full-time communication with the rover during its atmospheric entry, descent, and landing regardless of the landing site. The TEG developed for this mission allowed the rover to go further, travel more varied terrain, last longer, and power and heat a larger and more capable scientific payload compared to the solar-power alternative NASA had studied.
Developed in the US for multiple space missions, the radioisotope thermoelectric generator (RTG), or ‘space battery’, operates the rover’s instruments, robotic arm, wheels, computers, and radio. It is fuelled with plutonium-238 that gives off heat as it naturally decays. Obviously, few other applications are likely to use this as the heat source, but the principle of using TEGs for energy harvesting is transferable. No moving parts are required to convert this heat into electricity. The system uses thermocouples to create voltage from the temperature difference between the nuclear material and the cold Martian exterior.
The RTG provides a continuous source of heat, which is circulated through the rover by heat-transfer fluid plumbed throughout the system. It ensures that the instruments can be maintained within their operational temperature range. About 110 watts of electricity is generated continuously to power the rover's instruments, and is expected to continue for many years.
Predictive maintenance
Aircraft maintenance is a complex and costly business, which is beginning to exploit energy harvesting, and TEGs in particular, for a wide range of monitoring applications. Monitoring the external skin of the aircraft is important for the early detection of cracks due to wear, or damage caused by bird collisions. Autonomously-powered sensors can be installed in difficult to access locations and require no maintenance. TEGs can be used in series to power not only the sensors, but also the electronics required to transmit the sensor readings to a central location.
Lockheed Martin has been implementing energy harvesting systems for a decade or more on a number of aircraft platforms. One of its most recent projects is a predictive maintenance system deployed on its F-35 Joint Strike Fighter, and implemented as part of its Autonomic Logistics Information System (ALIS). ALIS enables daily operations of the F-35 fleet, including mission planning and flight scheduling to repairs and scheduled maintenance, as well as the tracking and ordering of parts.
Predictive maintenance systems are intended to help aircraft technicians anticipate impending engine problems and schedule maintenance only where and when it is needed, yet will help detect unforeseen faults or accidental damage. In operation, each F-35 will constantly monitor its own systems via Health and Usage Monitoring systems (HUMS) components, with data automatically relayed to the ALIS. Some of these self-reporting monitoring systems installed on and in the aircraft will comprise wireless sensor networks, some of which will be powered using energy-harvesting techniques, including thermoelectric generators.
Aircraft provide myriad temperature differential opportunities for the effective installation of TEGs. This could be the difference between the air temperature outside and inside the passenger cabin, or between turbine engine bearings and ambient air.
A major TEG vendor, Nextreme, has worked in partnership with aircraft OEMs to perfect its Thermobility TEG system, precisely for use in aircraft monitoring and predictive maintenance types of application. With its partners, Nextreme has developed a turbine health management system enabling jet engine maintenance experts to detect, diagnose, and take action on problems in engine-bearing assemblies.
It uses bismuth telluride integrated circuit material in the TEG device itself to generate about 20 to 30 mW of power from the heat of normally operating turbine engine bearings. This is more than enough to power the network of embedded sensors.
Selecting a TEG
TEGs come in a wide variety of sizes and electrical specifications. The most common modules are square, ranging in size from about 10 mm to 50 mm per side. They are usually 2 to 5 mm thick. For selection, Linear Technology’s Application Note¹ recommends designers look for data on output voltage or output power versus differential temperature, but states that it is not always provided. Two parameters that generally are stated, however, are VMAX and IMAX, which are the maximum operating voltage and maximum operating current respectively.
A good rule of thumb when selecting a thermoelectric module for power generation purposes, the note explains, is to choose the module with the highest product of (VMAX • IMAX) for a given size. This generally provides the highest TEG output voltage and the lowest source resistance. One caveat to this rule is that the heatsink must be sized according to the size of the TEG. Larger TEGs require larger heatsinks for optimal performance.
UK-based Laird Technologies, a long-time specialist in thermal management, EMI shielding, and wireless antenna solutions, has developed a wide range of TEG and TEC devices for heating, cooling, and energy-harvesting applications. Recently acquired by Nextreme, the Laird devices are already integrated into system-level solutions for the military and aerospace industries, as well as being supplied to OEMs.
Laird’s eTEG series of thermoelectric generators are micro-scale-size devices with higher output power density. They are suitable for use in wireless sensors and battery charging applications. Solid-state reliability and no maintenance requirements make them suitable to replace energy storage devices or provide power in remote places where access to a power line does not exist.
Meanwhile, Laird’s HV37 eTEC features an IMAX of 0.9 A and VMAX of 7.7 V, while the HV56 eTEC is quoted with IMAX of 0.9 A and VMAX of 10.8 V. For applications requiring higher current capability, the CP Series may be better suited. The CP08-71035-506 is epoxy sealed, and features an IMAX of 2.1 A and VMAX of 3.7 V. The substantially larger CP14-66101-500 is a silicone-sealed device with IMAX of 8.5 A and VMAX of 14.5 V.
A US-based component company, CUI Devices, offers a range of Peltier thermoelectric modules to help OEMs meet the increasing thermal challenges generated by today’s advanced electronics. A popular device is the CP20351, offering an IMAX of 2.0 A and VMAX of 15.4 V in a silicone-sealed package. For higher-current applications, the CP85338 delivers an IMAX of 8.5 A and VMAX of 8.6 V.
Exploiting a TEG
Linear Technology points out that when using TEGs, the electrical energy generated is typically unregulated and does not always lend itself to be used directly for powering electronic circuits. In some applications, there may not be a continuous, uninterrupted source of power. Again, depending on the specific application, average output power may not be very high, possibly as low as 10 µW to 10 mW. In essence, such characteristics demand judicious power management if the source is going to be useful in powering wireless sensors or other electronics.
Enter the LTC3108 and LTC3108-1, a series of highly-integrated DC/DC converters ideal for harvesting and managing surplus energy from extremely-low input voltage sources such as TEGs (see Figure 3). The step-up topology operates from input voltages as low as 20 mV. The LTC3108 is functionally equivalent to the LTC3108-1 except for its unique fixed VOUT options.
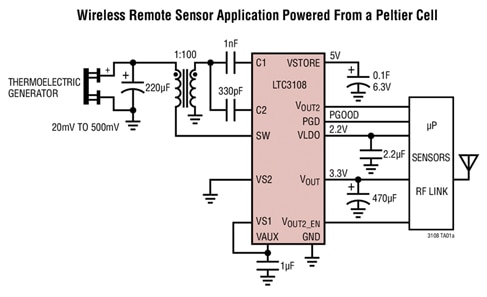
Figure 3: The LTC3108 DC/DC converter from Linear Technology is ideal for managing the thermoelectric energy generated by TEGs.
Using a small step-up transformer, the LTC3108 provides a complete power management solution for wireless sensing and data acquisition. The 2.2 V LDO powers an external microprocessor, while the main output is programmed to one of four fixed voltages to power a wireless transmitter or sensors. The power good indicator signals that the main output voltage is within regulation. The host can also enable a second output.
A storage capacitor provides power when the input voltage source is unavailable. Extremely low quiescent current and high-efficiency design ensure the fastest possible charge times of the output reservoir capacitor. This particular part is rated over the extended temperature range, -40 to 125°C, ideal for avionics and aerospace applications.
From an applications perspective, it is interesting to note that Nextreme's Thermobility solution, noted earlier, incorporates not only the Laird eTEG HV56 thermoelectric power generator module, but also the LTC3108 ultralow voltage step-up converter and power manager chip to provide up to 1 mW of electrical power (see Figure 4). It operates at temperature differentials as low as 15 to 20 K relative to ambient.
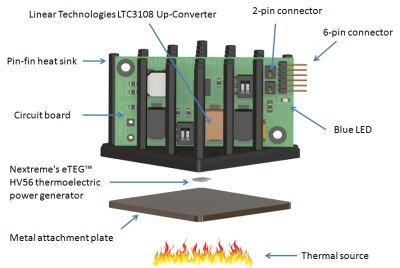
Figure 4: Nextreme’s Thermobility solution incorporating eTEG HV56 and LTC3108 to autonomously power an aircraft monitoring application to feed a predictive maintenance system.
Summary Exploiting waste heat to generate energy using thermoelectric modules is becoming more attractive for a range of applications, but especially in the military and aerospace sectors. These rugged devices enable sensors and their associated communications circuitry to be powered reliably and over the long term, without recourse to batteries. It will be fascinating to observe just how long the Mars Rover, Curiosity, continues to send back data from its sensors, powered by TEGs.
References:
- Linear Technology Application Note on thermoelectric applications
- US research (Northwestern University) into thermoelectric material
- IDTech report on Thermoelectric Energy Harvesting to 2023
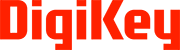
免责声明:各个作者和/或论坛参与者在本网站发表的观点、看法和意见不代表 DigiKey 的观点、看法和意见,也不代表 DigiKey 官方政策。