Smart Lighting Technologies Feature CFL Dimming, Wireless Control
投稿人:Convergence Promotions LLC
2012-03-06
Smart homes, the Smart Grid, and a proactive regulatory environment will ensure that lighting control over wireless links will remain a growth business over the next several years. More than 12 billion light bulbs are sold worldwide each year – most of them incandescent bulbs. Lighting accounts for up to 25 percent of home energy and a greater percentage in some commercial applications. Public utilities and governments in many countries are offering incentives to replace incandescent bulbs with compact fluorescents (CFLs). In the U.S. and Europe, government bodies have also enacted legislation prohibiting the use of incandescent bulbs by certain dates.
In addition to consuming far less energy than incandescent bulbs, CFL- and LED-based lamps typically last longer. The price of CFLs, in particular, is becoming competitive with incandescent bulbs due to generous subsidies and higher production volumes.
With the use of low-power wireless networks, lamps can be turned ON or OFF and even dimmed remotely, which opens another important door to intelligent lighting automation.
Wireless control reduces overall system cost by eliminating the cost of cabling. There is also a significant return on investment from energy savings obtained by intelligent control, especially when the wireless network includes sensors.
Two types of sensors are particularly useful: motion sensors that turn the lights ON or OFF depending on room occupancy, and photo-sensors that monitor ambient light from the sun. When ambient light is high, artificially-generated light (and its power consumption) can be reduced by 10 to 15 percent without the room’s occupants noticing.
Dimming over distance
Dimming CFLs will be an important feature in the future of smart lighting, and this topic will be discussed in detail later in this article.
The marriage of wireless IP connectivity with energy-efficient lighting technology in secure, compact, low-cost solutions can be implemented with modules small enough to fit in the base of a CFL. The modules consist primarily of a CFL controller, a power supply, and a wireless connectivity chip, which in turn often integrates a low-power radio, MCU, Flash, RAM, and a few necessary peripherals. A frequency crystal and decoupling capacitors complete the silicon components in the hardware design.
Power consumption is always a consideration in RF network designs and this is no less so for the CFL control circuit. Although CFL lamps are typically thought of as running cool – and they do as a system – the driver and control electronics can create a high-temperature environment inside the bulb, which means the ICs should be specified to operate at 125°C – and higher in some cases. The radio, power supply, and controller are the major contributors to power consumption, and each has important design considerations.
With wireless control, the radio chip is in standby mode even when the light is OFF. When the light is ON, the radio is always in receive mode, although it can be duty-cycled part of the time. The power supply can consume a substantial amount of energy and must be carefully chosen. The lighting driver’s efficiency is also important because the lamp must meet the minimum lumens per watt specification.
In addition to the silicon, a balun, an antenna, and software are needed to make a complete solution. The balun has two implementations: PCB microstrips, or a network of passives. Antennas can be fashioned from PCB traces, wires, whips, or chips.
Dimming circuit fundamentals
The majority of today’s residential and commercial use cases for dimming CFLs will default to manual dimming control as a baseline and then require adjustments to implement dimming with wireless control. In these cases, the wireless interface will be accompanied by a conventional, wall-mounted triac dimmer. The control and dimming circuits inside the bulb will respond to information transmitted from the triac dimmer after being actuated by either manual or wireless commands.
The design concept described in this article is, therefore, implemented using a standard, commercially-available wall dimmer and a ballast controller capable of dimming the CFL. The power supply is also an important component of the design. For 20 watt bulbs and above, external MOSFETs are required. N-channel, high-voltage MOSFET converters with specs including a source-to-drain voltage of 650 volts, continuous drain current of 4.5 amperes, and a power rating of 50 watts are suitable for this design. Infineon Technologies’ SPB04N60C3, for example, meets these criteria. Similar products that meet or exceed these basic specs include STMicroelectronics’ STD8N65M5 and Toshiba America Electronic Components’ TK6A65D.
Not all ballast controllers offer dimming functionality. NXP and STMicroelectronics both offer dimmable controllers. However, since this example targets a 20 watt CFL, the ballast controller design used is the UBA20270 from NXP Semiconductors and an external MOSFET.¹ STMicroelectronics’ L6574D013TR also supports dimming. The topology includes a Voltage Source Charge Pump (VSCP) that provides the necessary hold current for the triac in the dimmer. Without the VSCP, the CFL would not be continuously energized. Among other negative effects, such as shortening bulb life, this would also cause the bulb to hum during dimming. Without additional circuits to correct the problem, CFL loads would only be energized during the last part of each power-line half cycle.
Triac dimmer
The triac dimmer shown in Figure 1 employs forward phase-cut dimming.
Figure 1: Triac dimmer circuit (Courtesy NXP Semiconductors).
In Figure 1, the capacitor C (47 nF) is charged using a fixed resistor R (2.7 kΩ) and a variable resistor Rvar (470 kΩ), which sets the phase-cut dimming angle.
When the resistance is low, the capacitor charges quickly. As the voltage across the capacitor reaches the diac break-over voltage, the triac fires and the current Itriac flows. The current in the load must be as high as the triac latching current within the period the gate of the triac is fired or triggered. This current continues to flow until Itriac drops below its minimum hold current IH.
If the triac latching current is not reached by the end of its gate trigger pulse, multiple triac firing can occur in every half cycle. Multiple firings generate unwanted audible noise while the lamp is dimmed and should be avoided.
When the load is an incandescent lamp (resistive load), there is no phase shift between lamp voltage and lamp current. The current can rise high enough within the trigger period of the triac.
CFLs, on the other hand, cannot provide this level of current because of the capacitor in the input filter and the buffer capacitor. The triac is a bidirectional device that operates in two quadrants. During the negative half cycle, the identical process is repeated.
In this application, the LC filter can produce ringing of the triac current when the triac latches after a step response. The triac ringing current must remain above IH ensuring the triac remains powered up.
Triac wall dimmer with VSCP and CFL ballast
The circuit shown below corrects these problems. Figure 2 has a triac wall dimmer with the addition of a VSCP, CFL ballast controller, power supply, and miscellaneous control circuits. The half-bridge MOSFETs in the circuit switch the resonant tank circuit. The lamp voltage generates the triac boost or hold current.
Figure 2: Dimming circuit including triac dimmer, voltage charge pump, and CFL ballast (Courtesy NXP Semiconductors).
The VSCP maintains triac conduction even at deep dimming since the minimum holding current is exceeded for low phase-cut angles. A minimum hold current of 30 mA to 50 mA is sufficient for most triac dimmers. The VSCP operates in discontinuous mode and the average input boost current follows the input mains voltage.
The dimming control signal for the DCI pin is derived from the average of the mains rectified signal. This control signal decreases during dimming and simultaneously the frequency regulation loop of the IC increases the frequency of the half-bridge. These actions ensure the voltage on the CSI and DCI pins are equal at 0.34 volts.
Design considerations for a low-power RF (LPRF) control system
Wireless control enables remote human intervention over the Internet. Smart phones, tablets, remote-control devices, and actions initialized by a utility over the Smart Grid can all be employed to reduce lighting costs. IP connectivity may influence the choice of the wireless protocol that the design team selects. Low power consumption enters into the design decision and, in many cases; the protocol’s scalability can be an important design decision.
IEEE 802.15.4 specifies the PHY and MAC layers for low-rate wireless networks (LR-WPANs).² It is a well-known standard with widespread industry support and an abundance of silicon implementations. Its proven track record in applications such as consumer remote controls (RF4CE), metering (ZigBee Smart Energy), and industrial control (Wireless HART) make it the clear favorite to dominate the PHY/MAC market. On the protocol front, 6LoPAN and ZigBee are leading contenders for wireless lighting control. Both are open, low-power radio standards that support IEEE 820.15.4.
For lighting applications that will include IP connectivity, 6LoPAN has the advantage over ZigBee of being IPv6-based, which greatly simplifies gateway design and speeds software development and maintenance for designers already familiar with IP interfaces and sockets. It is basically a header compression scheme for IPv6 packets defined by the Internet Engineering Task Force (IETF) and promoted by the Internet Protocol for Smart Objects (IPSO) Alliance. A significant engineering decision consideration for designers is the fact that the smaller packets used by 6LoPAN require only small memory footprints. Memory size influences cost and energy consumption, but in this case footprint is additionally significant because the control module is embedded in the base of a bulb.
Mesh or tree topology?
6LoPAN implementations require the addition of an addressing and routing protocol, such as would be specified for a mesh topology or a tree topology.
Although mesh networking might be the intuitive choice because it has often been associated with 802.15.4, adopting a tree topology has the advantage of better scalability. Because the tree topology has a root node that is aware of all other node addresses (in the lighting use case, nodes are principally bulbs and sensors), it can scale to 500 nodes in a single network without negatively impacting performance. The same cannot be said for a mesh network, in which nodes communicate directly with each other (peer-to-peer). This communication scheme can lead to congestion in a large network.
On the other hand, 6LoPAN is a relatively new standard that does not yet have standard-based solutions for commissioning and security (implementations by companies can include them, of course). It also lacks an application-layer specification that is analogous, for example, to the ZigBee® Smart Energy profile, which could lead to interoperability problems across vendors.
ZigBee is a well-established standard and as such has at least one important advantage over 6LoPAN – it offers application-layer profiles that can reduce development time. Profiles also help ensure that radios from different vendors used standard commands and provide standard commissioning and security capabilities. The ZigBee Alliance has promulgated profiles for home automation and building automation but has not, as yet, settled on a lighting control profile.
MCU/Transceiver chips
For the majority of residential and commercial lighting applications, a single chip that integrates both an 802.15.4 radio and an MCU is the most cost-effective solution. Many MCU/radio chips are available, including NXP’s JN5148, which integrates the radio with a 32-bit RISC processor, 128 kB Flash, and 128 kB RAM. Of the numerous others, Ember’s EM351-RTR has the same integration with the radio: a 32-bit RISC processor and 128 kB Flash and 128 kB RAM. Freescale Semiconductor’s MC13224VR2 integrates its 32-bit RISC processor with 128kB Flash and 96kB RAM.
Taken together, the possible combinations of networking protocols and MCU/radio options can be intimidating. For lighting applications that are expected to be part of a growing network-of-things, however, several key parameters should be considered. First, if the network is expected to grow over time as more appliances, sensors, lights, and other devices are added, then it should support 250 to 500 nodes without a significant loss of performance.
Also, peak performance for the transmit current of the MCU/802.15.4 chip should be 20 mA or less. Memory requirements should be as low as possible since they have a major impact of cost and footprint – 128 kB Flash and 32 kB RAM are good targets. Receiver sensitivity is typically a decision point, and100 dBm should be sufficient for a smart lighting application, although some radios outperform that figure.
Summary
A robust market for energy-efficient lighting options coupled with wireless 802.15.4 modules small enough to fit in the base of a CFL will create a new market in smart lighting in homes and lighting automation in commercial and industrial buildings. Cost, power consumption, footprint, and dimming functionality are critical design considerations for market acceptance. While silicon and protocol stacks are readily available for the wireless component, silicon vendors have launched only a few ballast controllers that support dimming. This could be both a market advantage for those companies or a bottleneck in the acceptance of smart lighting.
References
- Application Note AN10961: Dimmable CFL using the UBA2027X family
- IEEE 802.15.2 standard
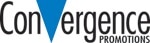
免责声明:各个作者和/或论坛参与者在本网站发表的观点、看法和意见不代表 DigiKey 的观点、看法和意见,也不代表 DigiKey 官方政策。