RF Power Measurement Techniques
投稿人:Convergence Promotions LLC
2010-12-22
With everything today going wireless, RF power measurement is rapidly becoming a necessity. This article highlights many useful techniques for measuring RF signal levels accurately in order to optimize the performance of these wireless systems. The article discusses the optimal approach for varying application requirements.
RF signals can take many forms, from a single carrier continuous wave (CW) to that of a multi-carrier, QAM (Quadrature Amplitude Modulation) that contains high crest-factor wave shape. Measuring the power levels of these widely varying signals requires understanding their characteristics as well as the required measurement accuracy. If the signal is bursty, such as that in a TDD (Time Division Duplexing) system, it becomes more complicated as there are time domain measurement considerations. In any event, selecting the right detector type can help simplify the design task.
Measuring RF power using peak detection
Take the simplest case of a CW waveform measurement. Even if the amplitude can vary, as long as the signal is within a prescribed time interval during which the amplitude is relatively constant, accurate measurement can be made with a peak level detector, such as the LTC5532 from Linear Technology. This device is built with a very fast Schottky detector with on-chip temperature compensation and a 2 MHz bandwidth output buffer. The internal Schottky circuit peak-detects the incoming RF signal and performs a peak-hold filtering, producing a DC output voltage that is proportional to the RF input peak level.
The LTC5532 is a very low power device that runs on 500 µA supply current in active mode. Yet its internal Schottky circuit is capable of detecting 7 GHz RF signals. A version of the part, the LTC5532EDC in a 6-lead, 2 mm x 2 mm plastic DFN package, offers low parasitics and can support operation to 12 GHz and higher.
Figure 1 shows this 12 GHz detector’s RF input matched to 11.5 GHz – 12 GHz. So its input circuit can be connected to the coupled output of a directional coupler or an RF source. The detector output amplifier gain is externally set by the R2 and R3 resistors at 10k each closing the loop around the internal amplifier with a non-inverting gain of two. At 12 GHz frequency, the circuit board material can introduce circuit parasitics that can affect the input impedance matching. However, acceptable performance can be achieved using standard FR-4 PC board material. The RF input matching consists of two 1.2 pF capacitors, C1 and C3. The C3 capacitor also serves the purpose of DC blocking because the device’s RF input is internally DC biased. RF input matching may need to be re-optimized for each specific application layout or other operating frequencies. At 12 GHz the RF input Return Loss measured 10 dB. Figure 2 on the next page shows a plot that depicts the detector’s transfer characteristic when a 12 GHz RF input signal is swept from -24 dBm to 8 dBm, its useful detection range.
Use a high dynamic range detector to measure low level RF signals
For applications requiring measurements of very low level RF signals, a high dynamic range detector with better sensitivity is necessary. Such a function is commonly used in a receiver that measures the RSSI for the purpose of providing AGC (Automatic Gain Control) feedback control. Other applications include field strength meter instruments. For this type of signal measurement, the log detector type is well suited since it measures the average power of a signal. Besides having high dynamic range and superior sensitivity, log detectors have excellent bandwidth characteristics extending to low frequencies. Their output provides a constant output slope in mV/dB log-linear scaling, facilitating ease of use.
An example of a high dynamic range log detector circuit is shown in Figure 3. The LT5538 is a log detector manufactured by Linear Technology that has more than 60 dB dynamic range. While the IC is capable of operating from 40 MHz to 3.8 GHz, the circuit as shown is designed and appropriately matched from 40 MHz to 2.2 GHz, covering a broad frequency range including all the cellular bands. The detector can discern a signal as small as -68dBm. Its dynamic range spans nearly 70 dB with an accuracy of +/-1 dB. At lower frequencies, for example at 880 MHz, its dynamic range improves to 74 dB.
Temperature drift is a difficult problem for high accuracy instrumentation as well as for many high performance wireless systems such as a cellular base-station. Typical desired accuracy is ½ dB or better, and held to this tolerance over the rated temperature extremes. The LT5538 achieves this desired accuracy over a wide dynamic range, thus minimizing the need for tedious calibration over temperature.
The LT5538 draws 29 mA supply current – necessary to achieve 4 GHz maximum operating frequency. The device has a shut-down capability. In sleep mode the device draws less than 100 µA quiescent current. The device can be turned on and initiates measurements in 300 ns. So this detector facilitates burst-mode measurements, conserving power in portable applications.
How to measure real power of high crest-factor signals
Modern broadband wireless data systems use complex modulation waveforms. For example, WiMAX and LTE (4th generation, Long Term Evolution) employ multiple carriers, each modulated with high-order QAM modulation. These RF signals have a peak-to-average ratio as high as 12 dB and are non-periodic in nature, making accurate measurements difficult. Calibration using look-up tables is often attempted with limited success to correct for simple modulated waveforms. However, with the trend of increasingly more complex modulation, correction using look-up tables is becoming inadequate.
A new RMS detector, the LT5581 from Linear Technology, helps solve these inaccuracy problems. The device employs an on-chip RMS measurement circuit that produces highly accurate power measurement of high crest-factor signals. It is capable of measuring signals from 10 MHz to as high as 6GHz. It has a dynamic range of 40 dB at lower frequencies and 30 dB at high frequencies. Additionally, the device offers exceptional accuracy over temperature, thus providing repeatable measurement. With all its capability, the device consumes a mere 1.4 mA supply current. The RF input is single-ended, so no RF balun transformer is required. Its wide bandwidth enables multi-band radios such as 3G or 4G broadband wireless data modem cards, 3G or 4G smart phones, WiMAX data modem cards and high performance portable radios.
The single-ended RF input lends itself well to directly tapping off from a RF source, such as a RF PA amplifier. An example of such an implementation is shown in a 5.8 GHz WLAN or WiMAX transmitter PA amplifier power control circuit in Figure 4.
The detector’s RF input is tapped into the PA output via a 20 dB resistive attenuator formed by the 604 Ω and 75 Ω voltage divider. This resistive tap eliminates the need for a directional coupler while saving costs. The 1.8 pF DC blocking capacitor serves to match the impedance of the detector. The entire resistive tap circuit introduces less than 0.2 dB insertion loss to the PA output, an amount that is quite modest.
For improved coupling accuracy, the 604 Ω and 75 Ω resistors should be 1 percent tolerance components and the 1.8 pF should be 5 percent or better. The recommended component values for the resistive tap are for reference. In actual implementation, the values may differ slightly, depending on parts placement, PC board parasitics and the parameters of the PA and antenna. However, using a directional coupler has the benefit of providing some directivity, whereas the resistive tap circuit does not.
That is, if the PA has excessive reflected power, a coupler would largely block that and will have minimum influence on the measurement accuracy. That is not so for the resistive tap circuit, which can contribute a small amount of measurement errors.
Figure 5 shows the detector transfer function as the PA amplifier output sweeps through the power range. At 5.8 GHz, the detector provides 25 dB dynamic range performance, typically sufficient for power control purposes. At lower frequencies, such as 2.1 GHz or 880 MHz, the LT5581’s dynamic range improves to 40 dB.
Conclusion
Depending on the signals to be measured, different choices of RF detectors are available to provide the optimum solution for the measurement needs. Schottky peak detectors are well suited for constant amplitude power measurements as long as the dynamic range is limited. Log detectors have higher dynamic range and superior sensitivity to measure low level signals. And for high crest-factor signals, RMS detectors produce the most accurate measurement results.
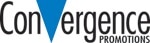
免责声明:各个作者和/或论坛参与者在本网站发表的观点、看法和意见不代表 DigiKey 的观点、看法和意见,也不代表 DigiKey 官方政策。