Modular Choices Simplify and Future-Proof M2M, Wi-Fi, and ZigBee Connectivity
投稿人:Convergence Promotions LLC
2012-04-26
The forecasts for machine-to-machine (M2M) connectivity identify tens-of-billions of devices exchanging data in medical, industrial, home, smart grid, and, as-yet-undefined, markets. Due to location, legacy retrofits, and geography, most M2M connections will be wireless, rely on IP packets and IPV6 addressing, and take place over WPAN ZigBee® or WLAN Wi-Fi® networks. As innovative M2M applications and wireless protocols evolve, modular one- and two-chip IC solutions are keeping pace to “future proof” designs. This article examines the features and system partitions of a number of ZigBee and Wi-Fi chipsets.
The Internet of Things
The “Internet of Things” describes a future where all manner of devices connect with each other and exchange meaningful data, increasingly with no human intervention. Assigning an address to every microcontroller-based subsystem will result in more uniquely connected nodes than anyone can count, but it’s surely in the billions in the next few years. A large percentage of these so-called M2M transactions will be IP-based and will occur in loosely-coupled, relatively close proximity 2.4 GHz and 5 GHz WPAN and WLAN networks dominated by ZigBee and Wi-Fi. ZigBee offers the benefits of up to 250 kbps data rates and extremely low power, while Wi-Fi boasts superior speeds up to 600 Mbps over already- or easily-installed consumer devices but at the expense of cost and power.
Yet the M2M growth phenomenon is characterized by this key fact: rapid change and evolution. No one knows exactly what systems or devices will be interconnected, or what kind of data will be exchanged. New systems and ideas are rapidly emerging, favoring modular approaches to chipset implementations. RF standards like 802.11ac (Wi-Fi) continue to evolve by adding channels, bandwidth, and security, while application-specific protocols such as ZigBee Smart Energy V2.0 are gaining traction in other venues such as powerline, automotive, and Wi-Fi.
For the reasons previously mentioned, chipset vendors are offering modular solutions for ZigBee and Wi-Fi M2M connectivity. In some instances, the MCU/MPU is separate from the RF section and connected by a standard UART or SPI interface. This allows swapping out either the processor or the radio or maintaining backwards legacy code compatibility. In other cases, a mixed-signal single-chip SoC is modularized or upgraded with freely available vendor protocol libraries and applications stacks. In this article, we’ll take a look at several IC manufacturers’ offerings and consider them in terms of board footprint, power consumption, RF performance, ZigBee protocols, Wi-Fi protocols, and available development tools.
Limitless recent M2M examples
The current red-hot growth of intelligent M2M is in areas like smart meters and the smart grid, home automation networks, medical, industrial (including factory floor and building energy controls), and much more. A smart choice is hedging one’s design bets with modular chipsets that offer wireless and software flexibility. The applications and market are growing so rapidly that no one can accurately predict what M2M systems will emerge, nor agree on the size of the market.
Research firm IDC, as reported by Microsoft, estimates the market for these and other intelligent embedded systems is 1.8 billion units and growing to four billion by 2015, roughly half of the entire embedded market as reported by IDC.1 Pike Research estimates there will be 535 million smart meters by 2015, 50 percent of which will use wireless mesh WPANs.2 Wi-Fi chipset supplier Redpine Signals and its partner Cypress Semiconductor estimated that there were more than one billion Wi-Fi shipments in 2011, helping to contribute to growing overall wireless units shipped to 20 billion by 2020.3
These numbers all describe M2M systems that are transferring data, making decisions based upon that data, and adapting. These are not just simple remote control transactions in TV or TiVo remote (for that, ZigBee’s RF4CE protocol is ideal). Cisco’s vision of M2M ranges from the idealized “internet- enabled refrigerator,” to dairy cow health monitoring using technology by Dutch start-up Sparked, where each cow transmits a non-trivial (for a cow) 200 Mb of data per year (see Figure 1). The Smart Home, on the other hand, balances energy consumption and utility rates by communicating between wirelessly connected smart appliances and smart meter technology (see Figure 2).
Cypress envisions bringing sensors and MCUs to a wirelessly monitored hospital bed, reducing the patient-to-machine tethering to just IV lines by removing wired leads. Recent research by Hallym University in South Korea and Pennsylvania State have proposed a wireless M2M solution4 to the vexing problem of locating items in unfamiliar retail stores using a combination of a Wi-Fi-equipped smartphone or kiosk that shows the item’s in-store location, coupled with product RFID tags, ZigBee mesh network, and ZigBee-equipped ceiling-mounted LED alert lights to make sure the shopper doesn’t miss the item.
The examples and potential of M2M used to be limited by the approximately 4.3 billion IPV4 addresses if they were inter-connected at all, but IPV6’s 3.4 x 10E38 addresses make imagination and business potential the only innovation constraints. The technology exists to realize just about any M2M implementation. The wireless challenge is to maintain flexible, standards-based, interoperable ZigBee and Wi-Fi chipset implementations that can be readily adapted to each current and future design scenario.
Changing standards
As Wi-Fi and ZigBee continue their popularity in myriad M2M, consumer, and business installations, changes and upgrades are planned – which is nothing new considering how often these standards have already evolved.
Wi-Fi is based upon IEEE 802.11, which has gone from the “a” revision up to the currently deployed “n” revision. Most of us were most familiar with 802.11b released in 1999 in the 2.4 GHz ISM frequency that at best offered 11 Mbps at about 38 meters indoors. It was affected by other RF home and business appliances and at 11 Mbps, was too slow for large file transfers or the erupting gaming and multimedia markets. While 802.11a offered 54 Mbps at 5 GHz, it was never very popular because the forthcoming 802.11g promised the same maximum 54 Mbps plus backward compatibility and interoperability (at the chipset level) with 802.11b. Chipset vendors migrated from 802.11a/b to 802.11b/g (with a few a/b/g offerings) and as the standards settled down between 2003 and 2009, Wi-Fi adoption in homes and businesses grew dramatically. JiWire reported a 425% growth in public hotspots between 2004 and 2009.5 Demand for more speed yielded 802.11n’s multiple channels, dual 20 or 40 MHz bandwidth, MIMO antennae, and rates up to 150 Mbps with double the typical indoor range to 70 meters.
Today the IEEE is working on the fifth generation 802.11ac called “5G Wi-Fi,” which rolls up the best of everything that came before: uncrowded 5 GHz operation, 80/160 MHz bandwidth, with rates in excess of 1 Gbps, eight MIMO streams, and backwards compatibility with a secondary 2.4 GHz transceiver. “Pre-ac” chipsets were announced at CES in January 2012.6 There are also discussions about new Wi-Fi mesh network protocols, allowing router-to-router, node-to-node and other data connection schemes that would certainly benefit M2M communications.
Similarly, ZigBee continues to evolve to benefit M2M applications. IEEE 802.15.4 defines ZigBee’s physical (PHY) and medium access control (MAC) layers and there have been multiple revisions that have added frequencies and modulation techniques on a per-country basis. RF spectra overcrowding, freed-up or government-grabbed frequencies, network topologies to enhance mesh connectivity, or security concerns could eventually force changes to the PHY and MAC layers, mandating IC chipset changes.
ZigBee is popular in M2M because it’s low cost and low power, but also because it is customizable for a variety of M2M systems. As shown in Table 1, ZigBee Smart Energy V2.0 is adding an IP-based protocol that might be ideal for many market segments besides the home automation network (HAN). As Smart Energy v2.0 gains traction, additional updates to the specifications may be required – obligating ZigBee chipset vendors to make hardware, firmware or software updates. As with Wi-Fi, modularity provides a flexible way of future-proofing an M2M system.
Partner Organizations |
HomeGrid Forum |
International Society of Automotive Engineers (SAE) |
IPSO Alliance (IP for Smart Objects; “primary advocate for IP networked devices”) |
SunSpec Alliance (renewable energy industry) |
Wi-Fi Alliance |
ZigBee Alliance |
Table 1: These organizations all support the ZigBee Alliance’s Smart Energy V2.0 profile which adds IP and other services.
Modular chipset benefits: ZigBee
Texas Instruments’ SimpleLink™ wireless initiative partitions wireless blocks into either single-chip network processors, or into RF transceivers – complete with network stacks and protocols – that can easily work with a designer’s favorite MCU or MPU. The mix-and-match modularity is no accident.
In the case of ZigBee, TI offers either single-chip SoCs with built-in MCUs and protocol stacks, or separate RF transceivers that are boltable to one’s favorite MCU or CPU, such as the MSP430 or Stellaris® ARM® CPU (see Figure 3). On the single-chip front, a SoC that combines an MCU such as an 8-bit 8051 with on-board peripherals such as USARTs, AES security engine, up to 256 KB of Flash ROM, 8 KB of RAM, 21 GPIO and a 12-bit , 8-channel ADC would be dense enough. But TI creates the mixed-signal CC2530 SoC by adding the 802.15.4 MAC, analog transceiver and TI’s Z-Stack™ (ZigBee/ZigBee PRO) or RemoTI™ (RF4CE) protocols (see Figure 4). Minimal peripherals are needed to get the CC2530 up and running.
Figure 3: Texas Instruments offers integrated ZigBee SoCs like the CC2530, or programmable RF transceivers with protocol stacks like the ZigBee Network Processor (ZNP) that can bolt to an MCU such as an MSP430 or Stellaris ARM (Courtesy of Texas Instruments).
TI’s CC2530 comes in a variety of SKUs, but broadcasts at 2.4 GHz in the ISM bands per IEEE 802.15.4 at a 250 kbps data rate. The CC2530F128RHAT SKU, for instance, consumes 28.7 mA at 10 dBm transmit, and 29.6 mA at a sensitivity of -88 dBm. The two to 3.6 volt Vcc sleep modes allow the device to exist for months to years on only battery power. At a svelte 6 mm x 6 mm in a QFN-40 package, the device can even be embedded in wearable health-monitoring M2M devices. Now that’s flexibility.
While an integrated, low-power single chip solution might be ideal in many M2M designs, it does “lock” the designer into that exact SoC configuration. TI also offers the CC2530ZNP, the ZigBee Network Processor version of the CC2530. This variant separates the network and application profiles so they can be run on a system processor such as an MSP430 MCU that talks to the ZNP via SPI using TI’s SimpleAPI™ and a mere ten software calls. For fast design spin-up, TI’s Mini Development Kit includes three target boards, two running on AA batteries and a sensor board, plus the IAR MSP430 KickStart™ compiler if one chooses that MCU.
Should the designer someday wish to change ZigBee application protocols from, for instance, Smart Energy 1.0 to the in-development Smart Energy 2.0, TI is likely to offer a Z-Stack update. Also, if a new customer requirement calls for adding ZigBee to a totally different CPU, having the RF section separate can be a definite advantage that allows upgrading a design.
Figure 4: TI’s CC2530 single-chip ZigBee SoC combines an 8051-based MCU with peripherals, ZigBee 802.15.4 MAC, RF transceiver, and even AES security (Courtesy of Texas Instruments).
Another variation on the single-chip ZigBee theme comes from Renesas in the M16C/6B3 and M16C/6B4 chips. Both are based upon Renesas’ wider-than-8051 16-bit M16C/60 core and differ in the amount of Flash memory and peripherals. Where the 64-pin M16C/6B3 has 256 KB of Flash and an 8-channel 10-bit A/D, the M16C/6B4 variant has 192 KB of FLASH and no ADC. Yet both devices are highly integrated, requiring minimal external components and are able to directly connect an antenna to the transceiver section (see Figure 5). Keeping with flexible modularity, Renesas offers AES and RF driver software, a network stack, and development licenses for ZigBee in addition to RF4CE should it ever be required, a nice modular option. Other interesting tools are the RF characteristic program that allows “tuning” a ZigBee design for maximum RF efficiency, along with a wide collection of development kits from the M16C series.
Figure 5: Renesas has two single-chip ZigBee solutions which vary only in the onboard memory and peripheral. The M16C series requires minimal external components but is available with multiple protocol stacks (Courtesy of Renesas).
But ZigBee M2M communication is defined by more than IEEE 802.15.4 PHY and MAC or ZigBee Alliance protocols. It’s also characterized by long periods of deep sleep where no data whatsoever is flowing between nodes and the network. One very interesting single-chip IC is Atmel’s ATmega128RFA1. Like the TI and Renesas devices, it’s in a small package (64-pad VFQFN) and requires minimal external components to send or receive on a ZigBee mesh. But the ATmega128RFA1 is notable for its wide Vcc (1.8 to 3.6 volts) and extremely low “picoPower” deep sleep power consumption.
At 3.0 volts, the 8-bit AVR-based device consumes a mere 250 nA while asleep, but keeps active Tx/Rx to under 20 mA. As well, Atmel includes protocols for BitCloud™ Smart Energy, ZigBee PRO, RF4CE, and even 6LoWPAN. Yet despite the chip’s high density peripherals and low power, it can scale up data throughput higher than ZigBee’s 250 kbps all the way up to 2 Mbps for non-standard M2M designs. Atmel also offers PCB-based ZigBee modules known as the ZigBit™ series. They use the ATmega® device or other MCU, an RF transceiver and combine an on-board antenna to dramatically shorten a design’s time-to-market. This kind of modular flexibility helps today’s product scale to tomorrow’s M2M system.
Wi-Fi – One chip or two?
At the chipset level, most IC vendors eschew the single-chip Wi-Fi SoC because of Wi-Fi’s complexity and constant evolution, instead choosing an implementation of the MCU or MPU plus Wi-Fi transceiver. TI’s SimpleLink concept is described on the company’s Wiki.7 It partitions the system between the MCU/MPU and the RF section, letting designers choose their favorite processor architecture such as the MSP430, ARM-based Stellaris family, or even a DSP.
With Wi-Fi, there’s also a large software burden due to the TCP/IP stack, security, driver for packet handling, and how it all plays with the OS. In a high-level OS like Android™ or Linux, these additions are handled well but place a huge load on an 8- or 16-bit MCU. TI’s solution? Besides dividing the hardware, the software is also balanced between the processor and radio. For example, the data throughput needs of an MCU-based M2M connection are less than a consumer tablet streaming Hulu: on the order of tens to hundreds of kilobytes per second as opposed to megabytes per second. So the network stacks are adjusted and the software split between the processor and radio transceiver.
TI builds its own radios for ZigBee, but currently works with third party vendors for Wi-Fi. An example of TI’s MCU/MPU and radio partition is the TMDSEVM3730 development module (see Figure 6). Designed to work with AM37x or DM37x ARM Cortex™-A8 type MPUs, the evaluation board uses an Laird Embedded Wireless Solutions 802.11 b/g/n chipset, DM3730 processor, DSP, 3.7-inch LCD touchscreen, and an SD card loaded with Android and Linux.
Microchip partitions its Wi-Fi (and ZigBee) chipsets similarly to TI’s SimpleLink by offering the MRF24WB0MA (built-in antenna) and MRF24WB0MB (external antenna) 802.11b RF transceiver solution. Designed to work with the company’s PIC18, PIC24, PIC32 and dsPIC MCUs, the 36-pin, 21 mm x 31 mm device has one and two megabytes per second data rates, built in AES security, a hibernate mode of 0.1 μA, and minimal external componentry. Microchip offers software and development tools galore, ranging from a free TCIP/IP stack to an 802.11 PICtail™ development board, originally from Microchip’s acquisition of ZeroG Wireless in 2010. The modularity of Microchip’s two-chip solution is ideal since the RF IC works with at least four different MCU families, allowing designers to future-proof their designs with modularity.
Other MCU vendors such as Atmel, Cypress Semiconductor, and Silicon Labs all have the ability to communicate via UART or SPI with third party, highly integrated RF Wi-Fi transceivers. Some, like Cypress Semiconductor, have announced partnerships and have introduced purpose-built demonstration kits that work with devices like PSoC® 3 and PSoC® 5 MCUs.
M2M modularity means future-proofing ZigBee and Wi-Fi
Wirelessly connected M2M-based embedded systems are poised for explosive growth with forecasts for billions of connected nodes. As the sky's-the-limit M2M landscape changes rapidly, the ZigBee and Wi-Fi chipsets enabling that growth need to be as flexible as possible, with modular hardware and software that evolves with the market and changing applications.
References:
- http://www.microsoft.com/Presspass/Features/2011/sep11/09-09IntelligentSystems.mspx?rss_fdn=Top%20Stories
- http://embedded-computing.com/freescale-connected-metering-medical-applications
- http://www.cypress.com/design-partner-solutions/rs-cy8c001-220x-80211abgn-wi-fi-expansion-board-kit-psoc-redpine-signals
- https://www.theengineer.co.uk/issues/january-2012-online/leds-and-radio-waves-could-help-locate-items-in-shops/
- http://www.jiwire.com/sites/default/files/JiWire_MobileAudienceInsights_Q309.pdf
- http://low-powerdesign.com/donovansbrain/2012/01/26/802-11-to-the-nth-degree/
- http://processors.wiki.ti.com/index.php?title=Wireless_Connectivity&DCMP=wtbu_ecs&HQS=Other+OT+connectivitywiki
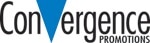
免责声明:各个作者和/或论坛参与者在本网站发表的观点、看法和意见不代表 DigiKey 的观点、看法和意见,也不代表 DigiKey 官方政策。