Technical Sports Clothes Get Smart
投稿人:DigiKey 欧洲编辑
2016-04-28
The year 2015 was widely hailed as the year that smart watches would kickstart the market for wearable technology. Certainly, dedicated fitness bands and activity-tracking apps for general-purpose smart watches have shown large numbers of consumers the scope of self-quantification: continuously recording data such as steps taken, calories burned and time spent above a threshold heart rate. Combining with location-based information such as GPS position, speed and heading from a smartphone can connect exercise activities with performance data for monitoring progress or sharing socially.
Smart watches could provide an effective introduction for a new generation of wearers to seek more detailed data about their performance and improvement. The next step is into more serious technical sportswear (Figure 1) that is capable of monitoring many more parameters and – with the right analytical apps – showing athletes how to improve their technique as well as fitness. Major sportswear brands and new startups are competing hard for leading positions in emerging markets for wearable sports technology.
Figure 1: Wearable technology all over the body can help measure and improve fitness and technique.
Vital Signs of Fitness and Performance
Wearable technology for athletes has been with us since before the 21st century, in products such as wireless heart-rate meters comprising a chest-strap sensor unit and separate wrist-worn display. These devices marked the advent of easy-to-wear, non-invasive monitoring of vital signs.
Today’s technical sportswear, including compression garments that are designed to aid circulation and muscle recovery, can provide a suitable medium for holding large numbers of sensors close enough to the wearer’s skin to pick up the tiny electrical signals generated by physical exertion. Many extra data types, in addition to heart-rate electrocardiogram (ECG) signals, can now be collected including electromyography (EMG) for analyzing muscle activity.
EMG takes advantage of the fact that muscles emit tiny electrical signals as they contract. Historically, EMG has been used in a clinical context by inserting small needles into the muscle tissue. Obviously this is impractical for use during sports and generally unsuitable for users who are not medically trained. Recently, surface EMG has become practicable, using sensors placed against the skin. Whereas needles capture highly localized data from within the muscle, surface sensors can give a more general view of muscle usage that can be analyzed to assess gait, style and power. Sportswear company Athos has used surface-EMG information to help customers achieve specific goals, for example working with a runner to achieve a significantly faster personal best performance over one mile by identifying and so helping to correct running style and symmetry issues.
In addition, accurate body-temperature monitoring is useful for monitoring fitness and can also protect the wearer against the dangers of over-exertion. TI has demonstrated that its LMT70 analog temperature sensor can fit inside a wearable device and measure body temperature to an accuracy within 0.1°C when in close proximity to the skin. The LMT70 is a low-power CMOS sensor accurate to within ±0.05°C from 20°C to 42°C, which covers the human body temperature range. It is packaged as a 0.88 mm x 0.88 mm 4-bump WLCSP, which allows the sensor to be positioned extremely close to the measurable heat source. The die on which the sensing element is fabricated is designed to be heated through the solder bumps, which must therefore be attached to a thermally efficient substrate. The LMT70’s wide operating voltage range of 2 V to 5.5 V allows use with all battery types commonly used in mobile applications.
Figure 2: The remote temperature probe is built for optimum thermal efficiency.
In TI’s reference design, the main body-temperature sensor is mounted on a small PCB that is separated from the main signal-processing circuitry so as to be placed as close as possible to the wearer’s skin. The circuit board incorporates thermal vias with copper pads to ensure body heat transfers quickly into the sensor for optimum responsiveness and accuracy. The remote board is attached inside a hollow metal cap using thermal epoxy, and electrically connected to the main signal-processing board using thermally efficient nickel wires (Figure 2). A second LMT70 is used for ambient temperature sensing, and the outputs from the two sensors are connected to two channels of an ADS1115 analog-digital converter (ADC). The block diagram of Figure 3 gives a high-level view of the signal path.
Figure 3: LMT70 reference design LMT70 reference design demonstrates body-temperature sensing in a wearable form factor.
Since the LMT70 has a transfer function of 5.19 mV/°C, the ADS1115’s 16-bit resolution is able to ensure accuracy to within 0.1%, which is the target for the project. The ADC communicates with the host MSP430G2553 microcontroller via I2C.
Sports scientists are also intrigued by the opportunities for measuring Galvanic Skin Response (GSR) using electrodes placed against the skin to assess athletes’ emotional responses to training routines. Widespread use of GSR in sports training may lie in the future, but Maxim has already produced a reference design for a wearable, mobile Galvanic Skin Response (GSR) system.
Maxim’s reference design is based on the MAX32600 wellness measurement microcontroller, which is connected directly to the skin electrodes and communicates with an Android device running the companion GSR/temperature-measurement app supplied free of charge with the reference design via a Bluetooth® Low-Energy controller connected to its SPI port. The microcontroller integrates an analog front end designed to support precision wellness measurements, so minimizing demand for external components such as a precision ADC like the ADS1115.
Analyzing Technique with Motion Sensing
Close-fitting sportswear is also ideal for embedding sensors such as MEMS inertial modules to monitor the wearer’s movements accurately. Form-hugging fabrics allow accurate sensing by helping eliminate noise that looser-fitting garments could introduce by moving relative to the athlete’s body. Sensing motion in multiple axes enables applications that are able to identify areas where technique could be improved, such as running stride or arm action. Some wearables are designed mainly with motion sensing in mind, such as wristbands or gloves to help improve technique such as tennis stroke or golf swing.
A number of products are already in the market, such as the PIQ, which monitors motion in 13 axes and communicates with a coaching app on the player’s mobile (Figure 4). The PIQ multisport sensor also incorporates GPS to provide extra functions for golfers, such as course information including distance to the hole.
Figure 4: A motion-tracking wearable such as PIQ can act as a virtual sports coach.
An inertial module such as the STMicroelectronics LSM6DS3 6-axis iNEMO® module combines a 3-axis accelerometer and 3-axis gyroscope in a single package, and has advanced features suitable for wearable applications. These include activity/inactivity detection and significant event detection, as well as built-in pedometer functions.
Recognizing the need for low power consumption in wearable applications, the module has an always-on low-power mode as well as a 0.42 mA low-power operating mode. The LSM6DS3 supports several operating modes including sensor hub mode, which allows up to four additional external sensors to be connected to the module’s master I2C interface. This can be used for connecting sensors such as a temperature or barometric pressure sensor to the system, using the LSM6DS3 as a hub. Figure 5 shows an example of how to configure the LSM6DS3 in sensor hub mode.
Figure 5: Sample code for configuring LSM6DS3 in sensor hub mode.
Conclusion
Tiny sensors and compression-fit sports fabrics are combining to bring about a dramatic change in the way athletes at all levels train to improve and compete. Together, they are able to transform athletic technical clothing into smart garments that can take on the role of personal coach.
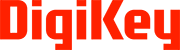
免责声明:各个作者和/或论坛参与者在本网站发表的观点、看法和意见不代表 DigiKey 的观点、看法和意见,也不代表 DigiKey 官方政策。