如何为物联网设备选择和应用天线
投稿人:DigiKey 北美编辑
2024-09-26
物联网 (IoT) 设备的激增不断加速和激发创新型终端产品的设计。但是,设计人员必须牢记,无论在硬件和软件上投入多少创意和精力,也无法改变天线的关键作用。如果天线不能正常工作,产品性能就会受到严重影响。
作为设备与无线网络之间的接口,天线是物联网设备设计过程中的关键部分。该器件可在发射器端将电能转换为电磁射频 (RF) 波,在接收器端将接收到的 RF 信号转换为电能。设计人员可以通过选择符合关键工程参数的天线来优化应用性能。然而,众多可用选项和考虑因素可能会导致设计周期延长和成本增加。
本文总结了天线在无线物联网设备中的作用,并简要介绍了影响天线选择的关键设计标准。随后以 Amphenol 的天线为例,说明了适用于低功耗蓝牙 (LE) 或 Wi-Fi 传感器、具有 GNSS 卫星定位功能的物联网资产跟踪器、Wi-Fi 接入点 (AP) 和 LoRa 物联网设备的选择。
解读规格书
天线的最终性能取决于工程决策,例如安装位置和阻抗匹配网络的设计。要想成功实施,需要仔细阅读天线的规格书。主要参数包括:
- 辐射模式:该参数以图形方式定义了天线如何在三维空间中辐射(或吸收)无线电能量(图 1)。
- 最大功率传输:当传输线阻抗 (Z0) 与天线阻抗 (Za) 匹配时,天线和接收器之间就能实现良好的功率传输。阻抗匹配不良会增加回波损耗 (RL)。电压驻波比 (VSWR) 表示传输线路与天线之间的阻抗匹配情况(表 1)。高 VSWR 值会导致高功率损耗。对于物联网产品而言,VSWR 低于 2 是普遍可接受的值。
- 频率响应:回波损耗 (RL) 取决于无线电频率。设计人员应查看规格书,了解天线的频率响应,确保在预定工作频率下 RL 最小(图 2)。
- 方向性:该参数衡量天线辐射模式的方向性质。最大方向性定义为 Dmax。
- 效率 (η):总辐射功率(TRP 或 Prad)与输入功率 (Pin) 的比值,计算公式为 η = (Prad/Pin) × 100%。
- 增益:该参数描述在辐射峰值方向上传输的功率大小。通常以全向天线为基准,用单位 dBi 表示。其计算公式为 Gainmax = η × Dmax。
图 1:以图形表示天线如何在三维空间中辐射或吸收无线电能量的辐射模式。规格书通常会显示天线按预期安装时在 XY 和 YZ 平面上的最大范围。(图片来源:Amphenol)
|
表 1:VSWR 表示传输线路与天线之间的阻抗匹配情况。对于物联网产品而言,VSWR 低于 2 是普遍可接受的值。(表格来源:Steven Keeping)
图 2:VSWR 和 RL 取决于频率。在预定工作频率下,RL 应尽可能小。(图片来源:Amphenol)
提升性能
性能不佳的天线会限制发射器端将多少电能转换为辐射能,以及接收器端从接收到的射频信号中获取多少能量。任何一端性能不佳都会缩小无线链路的范围。
影响天线性能的主要因素是阻抗。天线阻抗(与输入端的电压和电流有关)与驱动天线的电压源阻抗之间显著不匹配会导致能量传输不畅。
设计精良的阻抗匹配电路可使发射器电源的阻抗与天线的阻抗相匹配,从而将 VSWR 和随后的功率损耗降至最低。低功耗物联网产品的阻抗通常为 50 Ω。
天线的位置也会极大地影响最终产品的发射功率和接收灵敏度。对于内置天线,设计指南建议将其放置在物联网设备顶部的印刷电路 (pc) 板边缘,并尽可能远离运行期间可能产生电磁干扰 (EMI) 的其他元器件。但阻抗匹配元器件除外,因为此类元器件必须靠近天线。连接天线与其他电路的印刷电路板焊盘和印制线应该是限定净空区内唯一的铜导体(图 3)。
图 3:印刷电路板安装天线应靠近印刷电路板边缘放置。该天线还应远离其他元器件(阻抗匹配电路所用的元器件除外),并留出一定的净空区。(图片来源:Amphenol)
(有关天线设计指南的更多详情,请参阅如何在物联网设计中使用多频段嵌入式天线来节省空间、降低复杂性和缩减成本)。
天线类型
指定天线是物联网设备设计流程的关键部分。天线应针对目标无线接口的射频频段进行优化,例如,NB-IoT 适用于 450 MHz 至 2200 MHz 之间的多个频段,LoRa 适用于北美地区的 902 MHz 至 928 MHz,Wi-Fi 适用于 2.4 GHz 和 5 GHz,蓝牙 LE 适用于 2.4 GHz。
天线采用不同的电气概念。例如单极天线、偶极天线、环形天线、倒 F 天线 (IFA) 和平面倒 F 天线 (PIFA)。每一种都适用于特定的应用。
此外,还有单端天线和差分天线。单端天线是不平衡天线,而差分天线是平衡天线。单端天线会接收或发射以地面为基准的信号,其特征输入阻抗通常为 50 Ω。不过,由于许多射频集成电路都有差分射频端口,因此如果使用单端天线,通常需要一个转换网络。这种平衡不平衡转换网络可将平衡信号转换为不平衡信号。
差分天线则使用两个互补信号进行传输,每个信号都在其各自的导体中。由于天线是平衡的,因此当天线与带有差分射频端口的射频集成电路一起使用时,就无需使用平衡不平衡转换器。
最后,天线有几种外形尺寸,例如印刷电路板天线、芯片或贴片天线、外置鞭形天线和导线天线。图 4 显示了一些示例应用。
图 4:适用于各种物联网应用的不同天线。(图片来源:Amphenol)
匹配天线与应用
应用和产品外形尺寸决定了天线的最终选择。例如,如果物联网产品空间有限,则可将印刷电路板天线直接集成到印刷电路板的电路中。此类天线是 2.4 GHz 应用的绝佳选择,例如智能家居设备(包括照明、温控器和安保系统)中的蓝牙 LE 或 Wi-Fi 传感器。它们外形结构小巧,具有可靠的射频性能。尽管如此,印刷电路板天线的设计还是很棘手。另一种方法是从商业供应商处购买印刷电路板天线。然后可以使用背胶将其粘贴到印刷电路板上。
例如,Amphenol 的 ST0224-10-401-A Wi-Fi 印刷电路板印制线射频天线就是一种印刷电路板天线。该天线可在 2.4 GHz 至 2.5 GHz 和 5.15 GHz 至 5.85 GHz 频段提供全向辐射模式。天线尺寸为 30 x 10 x 0.2 mm,阻抗为 50 Ω。在这两个频率范围内,其 RL 均小于 -10 dB,在 2.4 GHz 频段,相对于全向天线,其峰值增益为 2.1 dBi,在 5 GHz 频段为 3.1 dBi。效率分别为 77% 和 71%(图 5)。
图 5:ST0224-10-401-A Wi-Fi 印刷电路板印制线天线在 2.4 GHz 和 5 GHz 频段中都很高效。(图片来源:Amphenol)
对于空间有限的物联网产品来说,另一种选择是芯片天线。自动化设备可直接将这种结构紧凑的元器件安装到印刷电路板上。该天线适用于基于蓝牙 LE 或 Wi-Fi 的无线物联网应用。芯片天线的主要优势是节省空间、制造成本更低和设计流程更简单。
如上所述,芯片天线的性能受到印刷电路板布局和周围元器件等因素的影响,但天线技术的进步造就了高效设备。芯片天线适用于从智能手机和平板电脑到智能家居系统和工业传感器等各种应用。
例如,Amphenol 的 ST0147-00-011-A 就是一款 2.4 GHz 印刷电路板表面贴装芯片天线。该天线可在 2.4 GHz 至 2.5 GHz 频段提供全向辐射模式(图 6)。天线尺寸为 3.05 x 1.6 x 0.55 mm,阻抗为 50 Ω。其 RL 小于 -7 dB,峰值增益为 3.7 dBi,平均效率为 80%。
图 6:ST0147-00-011-A 表面贴装芯片天线结构紧凑,在 XY 平面上可呈现全向辐射模式。(图片来源:Amphenol)
与印刷电路板天线一样,贴片天线也很小巧,可直接粘贴到印刷电路板上。该天线的典型应用是资产跟踪器或其他具有全球导航卫星系统 (GNSS) 功能的设备。GNSS 贴片天线由电介质基板上的贴片元件组成。高效特性可确保天线接收到来自多颗卫星的微弱 GNSS 信号。
例如,Amphenol 的 ST0543-00-N04-U 无源 GNSS 贴片天线可在 1.575 GHz 和 1.602 GHz 频段工作。天线尺寸为 18 x 18 x 4 mm,阻抗为 50 Ω。在这两个频率范围内,其 RL 均小于 -10 dB,在 1.575 GHz 频段,其峰值增益为 -0.5 dBi,在 1.602 GHz 频段为 1.0 dBi。效率分别为 80% 和 82%。
外置鞭形天线(如 Wi-Fi 接入点上的天线)安装在物联网设备外部,以优化无线电操作。外置鞭形天线可扩展信号范围、提高信号质量并克服障碍或干扰。此类天线适用于信号较弱或受阻的环境,例如家中的墙壁、天花板和家具会使信号衰减的情况。提供直式和螺旋式鞭形设计,每种设计都带有标准的射频接口连接,例如 SMA、RP-SMA 和 N 型。
例如,Amphenol 的 ST0226-30-002-A 2.4 GHz 和 5 GHz SMA 棒形射频天线。该天线是 Wi-Fi 接入点和机顶盒 (STB) 的理想解决方案。其可在 2.4 GHz 至 2.5 GHz 和 5.15 GHz 至 5.85 GHz 频段提供全向辐射模式。天线尺寸为 88 x 7.9 mm(直径),阻抗为 50 Ω。在这两个频率范围内,其 RL 均小于 -10 dB,在 2.4 GHz 频段,其峰值增益为 3.0 dBi,在 5 GHz 频段为 3.4 dBi。效率分别为 86% 和 75%。该天线配备 SMA 或 RP-SMA 插头连接器(图 7)。
图 7:用于 Wi-Fi 接入点的 ST0226-30-002-A 外置鞭形天线配备 SMA 或 RP-SMA 插头连接器。(图片来源:Amphenol)
对于 Sub-GHz 应用(例如在 868 MHz 频段工作的 LoRa 物联网设备)而言,螺旋式天线是一种既便宜又简单的选择。此类天线通常直接焊接在印刷电路板上,性能良好。不过,缺点是体积庞大,尤其是工作在低频的天线,而且与某些替代天线相比,效率相对较低。
例如,Amphenol 的 ST0686-10-N01-U 862 MHz 射频天线(图 8)。该螺旋式天线的工作频率为 862 MHz 至 874 MHz,阻抗为 50 Ω。天线采用通孔焊接安装,最大高度为 38.8 mm。其 RL 小于 -9.5 dB,峰值增益为 2.5 dBi,平均效率为 58%。
图 8:ST0686-10-N01-U 螺旋式天线是 LoRa 物联网应用的理想选择。(图片来源:Amphenol)
结语
无线物联网设备的无线电性能取决于天线的选择,因此设计人员必须从 Amphenol 等供应商提供的各种天线设计中谨慎选择,以最好地与应用相匹配。规格书在选择过程中至关重要,但遵循既定的设计指南可确保实现最佳无线性能。
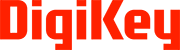
免责声明:各个作者和/或论坛参与者在本网站发表的观点、看法和意见不代表 DigiKey 的观点、看法和意见,也不代表 DigiKey 官方政策。