A Closer Look at Energy Harvesting: The Cymbet Energy Harvesting Development Kit
投稿人:Convergence Promotions LLC
2012-09-12
When bio-circuits start making their way into our products, we will need to feed them. Imagine feeding your iPad. Until then our technology depends on electrical energy supplied through lines, batteries, and generators.
But as T. Henry Morray stated, “The world is in a sea of energy,” and energy harvesting engineers are finally taking a closer look at energy sources around us that we can tap into. While people like Morray and Tesla pursued and demonstrated the evasive zero point energy, radiant energy, and vacuum energy that modern day science is just beginning to unravel for the masses, energy harvesting engineers are focused on more concrete and well understood commercially available sources like vibration, light, thermoelectric, and EMR/RF.
This article takes a look at the energy profiles of different types of energy harvesting sources and takes a look ‘under the hood’ at the Cymbet Energy Harvesting Evaluation Kit. This kit accepts energy from solar, thermal, EM/RF, and vibration sources. It then conditions that energy to charge the company's solid state rechargeable batteries, which in turn provide power when needed for our circuits.
The evaluation kit, solid state batteries, solar cells, thermoelectric modules, piezoelectric vibration transducers, as well as data sheets and training modules are available online at the DigiKey website.
Baseline of understanding
The different harvested energy sources have very different characteristics. As a result, a good understanding of them will let us design better ways to capture their energy without waste.
Photovoltaic solar cells are the most common and most widely deployed energy harvesting devices. They come in a variety of sizes and power ratings and provide a variable DC power level proportional to the incident radiant intensity at wavelengths to which they are sensitive.
Overall, three main types of photovoltaic solar cells are available, based on monocrystalline, polycrystalline, and amorphous crystalline materials.
Monocrystalline and polycrystalline cells are the oldest types and are based on silicon semiconductors in crystalline form. They implement the photoelectric effect based on the separation of hole and electron pairs when exposed to light (see Figure 1).
Figure 1: As electrons migrate to the electrode side, a net positive charge develops which allows current to flow.
Monocrystalline and polycrystalline photovoltaic cells can lose efficiency when shaded or partially shaded, but they work well even at higher temperatures, making them ideal for outside solar power installations.Amorphous photo cells are ideal for energy harvesting because they can be made very thin (less than 1 µM). In addition, they can be adhered to or deposited onto rigid metals or flexible plastics as carrier substrates. They use an irregular atomic arrangement (see Figure 2) allowing higher light absorption, thus generating more energy. Amorphous silicon photo electric cells also work better in partial shading, lending them to indoor applications, especially since they lose efficiency at higher temperatures.
Figure 2: The irregular shape of the amorphous silicon cell allows higher light absorption, letting these cells get very thin and even flexible.
Small discrete and serialized/paralleled wafers that we can wire directly into our applications are readily available off the shelf. For example, the amorphous AM-5610CAR from Sanyo is 25 x 20 mm and produces 5.1 volts at 2.4 mA steady current when fully illuminated. This is enough to run a small device and charge the battery at the same time.
Some of these cells can extract quite a bit of power. Take a look at the Sanyo AM-8702CAR. This discrete wire, flat, rigid amorphous photoelectric solar cell is serialized to put out six volts at over 17 mA. The –100 to +600C rating is wide enough for most outdoor applications as well as indoor equipment that takes its power from indoor lighting.
Do not totally discount crystalline cells for harvesting applications. There are some pretty neat little crystalline cells such as IXYS Corporation’s KXOB22-01X8, which is surface mountable and puts out an impressive 4.7 volts at 4.4 mA. Likewise, IXYS Corporation provides small monolithic surface-mount cells like the CPC1831N in an 8-pin SOIC. This part puts out an impressive eight volts but at a lower current of 25 µA (see Figure 3).
Figure 3: Small surface-mount encapsulated photovoltaic cells provide energy for low-power circuitry (Courtesy of IXYS Corporation).
While light is the most widely used form of ambient energy, others are starting to make inroads.
Vibration, shock, and impulse types of energy harvesting typically use piezoelectric transducers to convert physical molecular motion into electrical impulses that can be captured. The dynamic range is millivolts to hundreds of volts, so circuitry must be in place to capture and protect high voltage spikes.
Not every application can take advantage of shock and vibration to harvest energy, but applications such as truck chassis sensors, electromechanical factory machine sensors and actuators are constantly exposed to shock waves and/or steady high-level vibrations. Here piezoelectric sensors can be used. Take, for example, MIDÉ Technology Corporation’s V25W piezoelectric energy harvester. This flexible, flat-surface device is sensitive to vibrations in the 60 to 140 Hz range, enabling it to capture energy in real-time based on the deflection and distortion of the surface. This is ideal for 50 and 60 Hz rotating machines, for example, whose vibrations would otherwise be completely wasted energy.
Because of the AC and impulse nature of piezoelectric energy harvesting, rectification and filtering is needed, as is circuit protection since very high voltages can be generated from impulse shocks. The energy profile of shock and vibration are almost opposite that of solar cells (see Figure 4). With piezoelectric cells, the energy capturing and quantification are harder to nail down.
Figure 4: The energy from a piezoelectric harvester increases until it peaks at an operating voltage that is about half of its open circuit voltage (left image). Compare this to the energy profile of a standard solar cell where optimum power occurs when current is a percentage of short circuit current (right image).
With piezoelectric transducers you need to use tip mass, frequency tables, and curves from the data sheet in order to estimate the open circuit and operating voltages. Note that acceleration can also be used as a source of energy with piezoelectric energy sensors.
A piezoelectric energy harvesting training module on DigiKey’s website discusses techniques and circuits that can be used to transfer power from piezoelectric devices to circuits.
Thermoelectric sources for energy harvesting are not yet as popular as photovoltaic and piezoelectric, but they have a lot of potential. Two types of devices generate power from temperature changes: thermocouples and Peltier Effect devices. While both devices work on the principles of dissimilar metal junctions creating voltage potentials, thermocouples are used primarily for temperature sensors since they generate very low currents. They can be massively paralleled to increase their output, but to date this has not proven to be a cost effective solution.
Peltier Effect devices, on the other hand, are used mostly for heating and cooling applications, but the effect is symmetrical. That means as long as you keep one side cooled and the other side heated it will generate power that is proportional to the difference in temperature of the two sides.
Fortunately, Peltier Effect devices are available in a variety of sizes and configurations. For example, the smaller discrete 430290-501 from Laird Technologies provides asymmetrical sides that can handle temperature differentials of around 700C (see Figure 5). The larger CPM-2F assembly from CUI Devices is prefabricated to maximize heat absorption through aluminum plates bonded directly to the ceramic substrates.
Figure 5: Thermoelectric modules based on Peltier Effect devices are typically used for electronic heating and cooling, but since the effect is symmetrical they can also be used to extract electrical energy for temperature differences (Courtesy of Laird Technologies).
Thermoelectric devices are not yet widely adopted as energy harvesters, so data sheets do not usually provide detailed information for using them to extract electrical energy from the environment. These devices typically output higher currents at low voltages and require regulation and voltage conversions to be useful. They are emerging in hybrid solar tubes that act as glycol heaters in addition to generating electricity, so we should see more energy harvesting application information as time goes by.
A Peltier Effect devices training module can be found online at DigiKey’s website.
The last energy harvesting power source that is beginning to gain traction is RF/EM radiation that can be captured to power electronic circuits. While we have seen this technology used in a limited way with RF ID systems – which take RF energy from a reader and extract enough power to load modulate an output signal – the amount of energy captured is not yet enough to power even an energy efficient microcontroller and communications link.
However, near-field radiation systems like wireless charging systems can. An antenna is basically an inductor that acts as half of a transformer. A physically isolated transmitter radiates an AC field which the antenna can pick up, rectify, and use as a source of power. PC board pancake spiral antennas can be used to avoid more costly and heavy inductors (see Figure 6).
Figure 6: A PC board planar antenna can generate a radiated AC power field from which either an inductor or another PC planar antenna can pick up energy. This creates a low cost near-field power source for some energy harvesting applications.
While energy truly is around us all the time and can even be captured, what to do with it is where our clever pursuits can pay off. The effective power conversion, regulation, charging, and storage as well as the ability to supply captured power is where our design comes into play.
The underlying technology
Cymbet manufactures and sells solid state rechargeable batteries that can be surface mounted to a small PC board for low power, battery backup, RTC, and SRAM retention applications. The EnerChips, as they are called, feature flat and steady voltage profiles; high charge/discharge cycle life (typically 5,000); very low self-discharge rates (typically five years); and relatively fast charging (80 percent capacity in 30 minutes).
EnerChips are based on a new solid thin film that is fabricated as wafers and packaged just like an integrated circuit chip (see Figure 7). Standard solder reflow techniques can be used and there are no caustic flammable solvents that can leak, dry out, or catch fire. There are no special disposal requirements since EnerChips are fabricated with environmentally friendly materials. This also makes them well-suited for small, sealed designs such as wearable medical devices.
Figure 7: EnerChips are fabricated just like a standard IC without solvents and flammable electrolytes that can leak (Courtesy of Cymbet).
The EnerChips come in 12, 50, and 100 µA per hour ratings and with/without the company’s charge controller integrated into a single package. This means you can design your own charge/discharge energy interface controller or use Cymbet’s CBC915 EnerChip Energy Processor, which is basically a dedicated controller and sequencer that controls and monitors the batteries’ charge rate, discharge rate, and state. It also supports multiple batteries in series and parallel configurations to allow higher power capacities.
Because the batteries have such a flat discharge curve, it is hard to know how much energy they hold merely from a voltage reading. Active monitoring and gas gauging is needed to solve this. Cymbet’s CBC915 takes care of this.
If you are designing your own charge controller, you will need to implement a precise 4.1 volt charging regulator and monitor the discharge rates so as not to discharge them too rapidly. Too high a voltage, and the capacity degrades; too low a voltage and it won’t fully charge. In addition, close monitoring of the terminal voltage is needed, and a user’s design must remove any loading if the battery drops to 3.0 volts or less. This too can damage the battery. Again, the CBC915 takes care of this.
The output of the battery alone is rated at 3.8 volts without the embedded charge controller. When the integrated charge controller is used, the battery outputs a regulated and ready-to-use 3.3 volts – ideal for many of today’s designs.
The data sheets go into the specifics as to the ideal discharge rates for the batteries. This way you can know if you need to parallel more than one, especially if you are using pulsed moments of higher current draw (such as RF applications). Cymbet provides an application note that describes how to estimate battery life and design for pulse discharging applications like RF. Overall, the best battery performance and life occur when discharge rates are lower.
A kit is worth a thousand words
The small size and characteristics of the EnerChips lend themselves to low-power electronics, such as wireless sensors that can gather enough ambient energy from the local environment to run on their own. To demonstrate the use of energy harvesting to gather energy and charge the EnerChips, Cymbet has made available the CBC-EVAL-09 Universal Energy Harvester Evaluation Kit (see Figure 8).
Figure 8: The Energy Harvester Evaluation Kit has interfaces for solar, EM/RF, thermal, and vibration energy sources and contains the company’s charge controller chip (Courtesy of Cymbet).
DIP switch settings configure the board to accept power with basically five conditioning circuit interfaces: a high-voltage DC input, two high-voltage AC inputs, two low-voltage AC inputs, and a direct DC input (see Figure 9). Example circuits for the different energy sources include a boost converter, two charge pumps, and flyback converter. A solid state switch at the DC output allows the CBC915 controller to go from charging state to discharging state and back automatically when conditioned energy is available. There is also a low battery cutoff switch to protect the batteries which should not operate below three volts.
Figure 9: Bridge rectifiers convert AC to DC for both high- and low-voltage AC inputs; charge pumps, flyback, and boost converters are used to condition energy for the charge controller chip which also controls and monitors battery discharge (Courtesy of Cymbet).
Unpacking and Connecting
I received the CBC-EVAL-09 Universal Energy Harvester Evaluation Kit and unpacked the contents which included the main board, an example photo cell, a flat flex cable and a CD ROM. On the CD ROM were all of the data sheets, application notes, and manuals for this evaluation kit, as well as other evaluation kits Cymbet provides.
I like the 100 mil spacing pin headers since these provide an easy interface. Using those pins, I was able to connect my own solar cell to quickly test the board. I also liked the layout of the board – all energy conversion circuits are to the left and the energy controller chip is in center, with test points for every pin easily accessible for probing. The right side has the socketed EnerChip CBC51100 module which contains two of the 50 µA per hour batteries, one CBC050 rechargeable battery only and one CBC3150 intelligent thin film battery which contains a battery and charge controller. This provides a 3.3 volt, 100 µA per hour source that could be charged via energy harvesting and discharged with my test loads.
The DIP switches are of the miniature type so I needed good glasses to read the reference designators, but the manual also had large size prints of the switches, button, indicators, and connectors to which I could refer.
I liked how the status LEDs were not functional until a little switch button was pressed. This allowed me to see the operational status but not pull power away from the process just to light an indicator. The three status indicators let me know when the controller was tracking maximum peal power; when the holding capacitor was charging; when the EnerChips were charging; and when output power is being regulated. A nice feature is that the CB915 finds the maximum peak power point of the input transducer and indicates it has locked on.
I also liked the folded pin test points, to which I could easily attach a meter. This let me watch the battery voltage, capacitor voltages, and input voltages from my sources and loads.
With the supplied cell attached, I was able to watch as the 1.39 volt input was boosted to the 4.02 volts the EnerChips like to see (see Figure 10). The better regulated the charging voltage is, the better the performance you can expect to see from the EnerChips.
Figure 10: The test setup let me easily configure and connect various energy devices and evaluate the harvesting and charging technology offered by Cymbet.
When I tried the solar cell I lifted from one of my solar-powered garden lights, I was able to see that its output voltage was lower than the output voltage of the supplied solar cell. After a few seconds the EC LED indicated that even at the lower voltage the EnerChip was being charged, and after a few more seconds I saw the MPPT LED flash indicating that the controller had found and locked onto the maximum power point of the cell that I was using.
As the sun set, I saw that the rate of charge slowed, but the circuit was still extracting whatever energy it could and was still generating charge pulses to the battery, albeit at a slower rate.
I wired a thermoelectric device to a header and plan on testing this one as well as soon as I can build a test set that can control and monitor the temperature of two plates. This will let me characterize the energy available at different temperatures.
Cymbet’s solid state technology training module is available online at the DigiKey website.
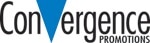
免责声明:各个作者和/或论坛参与者在本网站发表的观点、看法和意见不代表 DigiKey 的观点、看法和意见,也不代表 DigiKey 官方政策。